
May 26, 2022
Lorne Hofstetter has one of those curious minds that isn’t satisfied until he understands how something works, learns how to make it himself, and thinks of several ways to improve what he’s just built. Growing up outside of Boise, Idaho, on a small ranch as the middle child of three, he had plenty of opportunities for hands-on learning. But his engineering and physics-oriented mind really took off with remote-controlled sailplanes. “At 14, I was cutting my own wings out of foam and using equations I learned from library books to design model airplanes that were better for gliding,” he recalls. Whether slingshotting the gliders into the air with a giant rubber band or hucking them off of a mountain, he loved catching thermal uplifts of air and cruising in circles with the birds. These invisible currents would recur in another form when he started working on MRI technology.
Hofstetter traveled to the east coast to earn his undergraduate degree in Astronomy from Princeton. From there he landed a job at General Electric’s Global Research Center, in Schenectady, NY. The research center was unique in that it was less focused on a particular product line and instead contained many new technologies in one place – from energy storage in electric vehicles to the design of new jet engines. He saw a talk on MRI in 2008 that really intrigued him and shortly thereafter started working with the MRI team. Far from medical applications, one of the team’s projects was using MRI to validate the cooling channel geometries used in jet engine fan blades.
![]() Hofstetter at age 19, launching a glider from a mountainside. |
While working at GE, fate threw Hofstetter in the path of Dennis Parker, PhD, one of the senior researchers at the Utah Center for Advanced Imaging Research (UCAIR). The GE team was working on MR temperature mapping, something Parker had published several early papers on. Hofstetter read Parker’s papers and met him at the 2010 annual meeting of the International Society for Magnetic Resonance in Medicine. He also met graduates from Parker’s lab and others who spoke volumes about the influence Parker has on the field: Nick Todd, PhD, who works at Brigham and Women’s Hospital in Boston; Viola Reike, PhD, then at UCSF and now a part of UCAIR; Christopher Dillon, PhD, who worked with Dennis’s team and is now faculty at BYU; and many more. When Hofstetter decided to attend the MD/PhD program at Utah, research colleagues at GE thought very highly of Parker and several recommended that he work with him if given the opportunity.
Maturity and Patience
Yet Hofstetter had a curiosity about medicine that rivaled his desire to hit the ground running with Parker’s lab. So when he started his MD/PhD program at the University of Utah, he stuck with the dedicated track of two years in medical school training before starting graduate school research in year 3 in Dr. Parker’s group.
His patience speaks to his maturity – having already worked at GE for 7 years, published a paper on MR temperature mapping, and even started and sold an internet company with his wife before pursing his MD/PhD. “True to this mature start,” Parker says, “Lorne was a highly motivated, self-starting graduate student who was like sponge, absorbing everything that you and everyone else could give him.”
Despite his incredible contributions to science, Parker is humble enough to know when others in the room are as smart as he is. He saw a similar respectfulness in Hofstetter. “Lorne was always respectful when he realized he knew more than you did on a topic – he always gave you the benefit of the doubt if you offered information that differed from his,” states Parker.
Human Tissue Bounces, It Turns Out
When Hofstetter joined Parker’s lab, they were in the midst of improving upon a 10-year journey started by faculty member Allison Payne, PhD, to build the world’s first-ever noninvasive breast ablation system for women with breast tumors. This system uses focused ultrasound waves to “cook” the tumor tissue very precisely within the breast, all done within the MRI machine to measure location of the tumor and the amount of heat put into the tumor (see Surgery with Sound: Star Trek Medicine for Breast Tumors for more details). A large patient safety and quality question for this treatment was to make sure that doctors would know when the tumor tissue was fully neutralized, and Parker had received an NIH R03 grant to quantifying tissue stiffness during focused ultrasound treatments. That’s right – just like a steak gets stiffer when cooked, human tissue changes its mechanical properties when it is ablated as well.
This topic is closely related to MR thermometry, but attempts to directly measure the progress of focused ultrasound treatment in a way separate from temperature change. Hofstetter was very intrigued, and set his mind to the problem.
Their approach was to probe the tissue with a low-energy ultrasound pulse: “It’s like dropping a pebble in a pond,” Hofstetter explains, “and the MRI tracks the ripples. The speed at which the ripples travels tells you how stiff the tissue is.” This is also called the shear wave speed. The ultrasound pulse is low enough energy to not burn the tissue, just make it bounce.
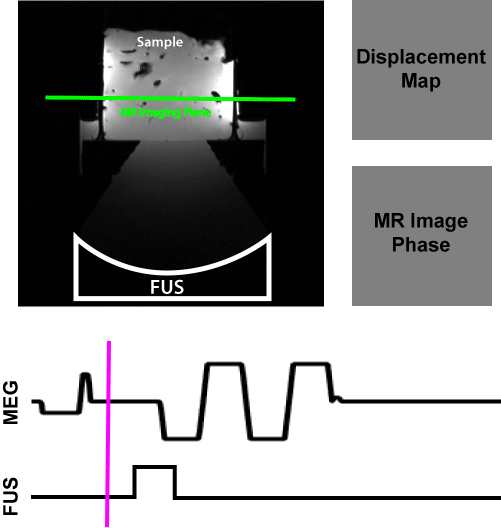
From one of Hofstetter's publications, this video shows how they encoded the ultrasound pulse and the resulting shear wave propagation measured in the MRI machine.
![]() This figure shows Hofstetter’s tissue stiffness measurement in an ex vivo liver sample (as well as MR thermometry before and after tissue ablation). The top left image shows the tissue stiffness before treatment. Top right shows the tissue stiffness following treatment. Bottom left is the peak temperature reading and bottom right is the thermal dose (this ablation area is outlined in the top right image). Within the thermal dose boundary, the mean shear wave speed increased from 1.65 to 2.52 m/s after ablation. That means we’ve got some much stiffer tissue. |
Other researchers have showed that MRI can measure an ultrasound pulse sent into tissue, but MRI is notoriously slow in scanning the information. In the case of this prior research, it took 20-30 minutes to generate a tissue stiffness map using this approach. But Parker and Hofstetter created a traveling wave with the ultrasound to do this work while the MRI was scanning. They managed to create a two-dimensional map of tissue stiffness in an astonishing 10-second acquisition. Finally, this technique was fast enough to be considered for real-time patient treatments.
They wanted to make that patient application a reality, so Hofstetter set about some additional research and publishing a few more papers. They got down to some serious math and validated a mathematical model of how shear waves propagate through tissue. With this model in hand, they were able to explore, develop, and evaluate ways new ways to use the MRI to extract the tissue stiffness information. They also validated with human tissue “phantoms” (models) that the ultrasound device used in the treatments and the MRI machine could work together in a fully simulated scenario.
These extra steps reveal the true attention to translating basic science into medicine that happens at UCAIR. Hofstetter feels very good about this work that resulted in his PhD. “We really explored the solution space for this problem,” he reflects. “We proved that we can accurately model it and test it from multiple perspectives.”
Getting Sidetracked is Sometimes Great
One of the reasons Hofstetter was excited about the work in Parker’s lab was the environment at UCAIR where custom hardware was being built just steps away from the laptops and whiteboards where the math and physics were being worked out. “I had done MR pulse sequence programming and image processing work during my time and GE, but I really wanted to learn more about hardware and building hardware.” During his time at UCAIR he got the opportunity; he learned to model, build, tune, and match MRI coils in the Coil Lab. The work wasn’t strictly related to his tissue stiffness project, but it’s where his passion and curiosity drove him. Thankfully, Parker is the type of supportive lab leader who encourages this in his go-getter lab members.
“I guess my advice to graduate students is that while you need focus in grad school, it’s OK to have little passion projects,” Hofstetter says. “Of course, only if your advisor is ok with it!” he adds.

One Last Idea to Plumb
There’s something of that teenager – finely controlling little servo motors on the flaps of his custom plane, eyes tracing its circular path in the sky, eking out as much height and soar that the thermals had to offer – that still resides in Hofstetter and probably always will. And it’s that early fascination with motors and engineering solutions that gave him one last inspiration before parting ways with Parker’s lab.
Once again, his focus was patient interventions but this time surgical procedures that require a precise movement, like a biopsy. MRI is an excellent visualization tool for soft tissue and very sensitive areas like brain tissue, but it is not widely used to monitor procedures like biopsies for several reasons: it has a huge magnetic field that limits what type of equipment can be used, the imaging time can be long, and it is difficult to access the patient because they’re inside the bore of the machine. But what about a robotic tool small enough to be used next to the patient? This type of robotic solution might lead to safer procedures and more precise targeting because the imaging and the procedure could be done seamlessly, and even at the same time.
“A typical robot has things that need to be moved and an electromagnetic motor is often used to move them,” Hofstetter explains. “Conventional motors are full of steel and magnets which are inherently incompatible with MRI.” Even if you could make a motor out of completely non-magnetic parts, the electrical noise generated by the motor would interfere with sensitive equipment in the MRI, leading to poor image quality.
“People have tried using piezo-electric actuators and pneumatic or hydraulic motors outside of the room with long tubes and big equipment or long mechanical wires,” he states. This is far from ideal and robotic system research in MRI has been flummoxed by the incompatibility of conventional motors.
But inspiration has a way of growing out of seemingly insignificant things. “I saw a talk at a conference three years ago,” he recalls. “A group was trying to transfer power wirelessly from an MRI machine to a receive coil on the patient.” Their goal was to avoid a bulky network of wires connected to a separate power source. “This talk reminded me that the MRI machine already has most of the components of a motor.”
Hofstetter sat on that initial thought for a year or two, but came back to it and started to work on an MRI motor in his last year with Parker’s lab. The concept was ingeniously simple: use the main magnetic field produced by the MRI to power a motor made out of non-magnetic materials. After refining the initial concept, he built a simple system using his motor to move a biopsy needle within an MRI machine, piggybacking on the MRI’s magnetic field to work the motor. He and Parker thought that there would be some degradation of image quality, but after several design iterations and input from our Coil Lab director, Rock Hadley, PhD, they solved that problem, too. Hofstetter and the team had developed an entirely new way of safely controlling a robot inside an MRI and potentially a new way to do procedures under real-time MRI guidance.
Parker is extremely pleased with the evolution of Hofstetter’s work. “The MRI motor is an incredibly unique idea,” he declares. “It has consumed his spare time (after receiving his PhD) and has totally demonstrated feasibility.”
They have recently published a paper in Nature Communications Engineering on their initial work. Parker and the Pivot Center at the University of Utah immediately set to work helping him make this idea something that can be built into new patient care scenarios. They have filed several provisional patents, and Hofstetter is leading the efforts at a new company setting out to refine the foundational technology behind this new surgical paradigm: 4D Surgical. The name is a nod to the fact that they can monitor a 3D space in real time (hence, “4D”) during a robotically controlled surgical procedure.

The U of U Radiology and Imaging Sciences Difference
Hofstetter is clear about how grateful he is to have pursued his graduate work in our department. “I was very happy with how open the community is here at Utah,” he says. “I reached out to a breast radiologist faculty member, Huy Pham, and he invited me to shadow him on a procedure. This was instrumental in helping me to design a first proof of concept robot.”
He adds that having Dennis Parker and Rock Hadley, the director of the Coil Lab, jump in to help brainstorm and troubleshoot was fantastic. Also, he credits Robb Merrill in the Coil Lab for doing all of the 3D printing for the motor parts. In short, Hofstetter recognized that we have the technical excellence and breadth of knowledge at UCAIR and the clinical partners in the health care system to help show us where the technology would be useful.
Hofstetter is excited to get back and finish medical school, but only after taking a 1-year leave of absence to see how far he could take the technology. “A few weeks away from a planned return to medical school, I was too excited about the progress to leave it where it was,” he laughs. He’s working on several SBIR grants to support 4D Surgical’s collaboration with the experts at UCAIR to make that new surgical paradigm a reality. “We want more control and more degrees of freedom in the robotics,” he says.
As parting words for budding graduate students, medical students and entrepreneurs, Hofstetter says, “It’s not a sprint. You’ll likely need to overcome a lot of barriers to become successful, and this will take some time.”
On the other hand, he’s open to the fact that if they see some quick wins and get past some technical hurdles, it would make sense to put this part of his career into the company.
Wherever he lands, we’ll be happy to collaborate with Lorne Hofstetter, PhD. He’s a great case study in what a motivated and curious graduate student can do at UCAIR. And if that praise isn’t enough, Dennis Parker states. “Lorne reminds me of the self I would have liked to have been.”
Satoshi Minoshima, MD, PhD, the Anne G. Osborn Chair and Professor of Radiology and Imaging Sciences, adds his support as well: "Lorne has accomplished much on his way to his PhD. He is a great talent and wonderful role model for future physician scientists."
About the Author:
Michael Mozdy, MA, is the Associate Director of Science Communications for the Department of Radiology and Imaging Sciences.